Research directly converts embryonic fibroblasts into laboratory-induced neurons
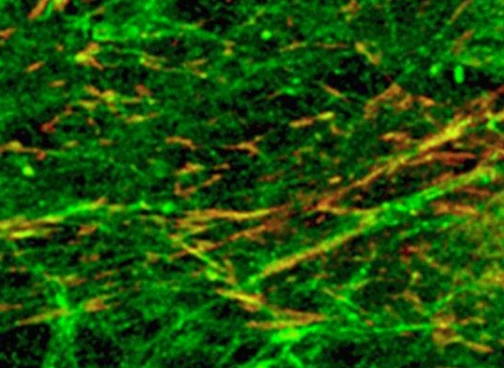
Research directly converts embryonic fibroblasts into laboratory-induced neurons
Copyright © iCell Bioscience Inc, Shanghai 2018-2019
The human brain is made up of highly complex and extensive networks of cells and neurons, yet the existing scientific understanding of the developing brain is relatively limited. As an evolving field, neuroengineering uses advanced techniques to manipulate neurons. Scientists in this discipline are able to develop disease models of the central nervous system and the peripheral nervous system to understand neurological diseases and to build the next generation of biological materials for neural tissue engineering. The ability to directly reprogram mature cells such as fibroblasts (a somatic cell) and convert them into neurons also presents a good therapeutic potential. Neuroengineering techniques aim to design and construct a wide range of applications to regulate neurons.
Neuroscientists have recently demonstrated that brain extracellular matrix (BEM) after decellularization of human brain tissue can simplify the process of directly converting primary mouse embryonic fibroblasts into laboratory-induced neurons (iN). iN cells produced on BEM were transplanted into the brain of an ischemic stroke animal model, resulting in a significant improvement in motor behavior. The study also demonstrated that the combination of a three-dimensional BEM hydrogel with a microfluidic system can reconstruct the brain-like microenvironment, which promotes direct reprogramming and neuronal transformation more efficiently, thereby facilitating animals to undergo function after undergoing cell transplantation. restore.
During neuronal reprogramming, this constructed three-dimensional system is thought to stimulate YAP protein and histone modifications for mechanically sensitive signal transduction. This three-dimensional BEM hydrogel may also provide microenvironmental memory to maintain the functional phenotype of iN cells obtained during this transformation.
In this study, the researchers first extracted the extracellular matrix of the brain from human donor brain tissue and subsequently constructed a soluble mixture of brain-specific natural components. This soluble mixture is then used to develop and test two-dimensional surface coatings or three-dimensional systems to culture fibroblasts that undergo direct neuronal programming in the laboratory.
The use of fibroblasts to transform into neurons in this study, in the presence of tissue-specific transcription factors, can directly convert mature somatic cells into different cell types, a process known as transdifferentiation. Unlike typical viral delivery systems, plasmids are used as biomedical delivery vehicles, and neuronal tissue-specific elements, including BAM factors, are transfected into fibroblasts by electroporation to induce neuronal transdifferentiation.
This study demonstrates that BEM significantly accelerates direct neuronal reprogramming because iN cells display electrophysiological function and marker expression of mature neurons as early as day 14 under laboratory cell differentiation conditions. Immunocytochemistry and genetic analysis have shown that BEM improves growth and differentiation compared to conventional biomaterials such as laminin and fibronectin, which are often used to make two-dimensional extracellular matrix (ECM) coatings.
After extensive gene expression profiling and electrophysiological testing in the laboratory to characterize iN cells, scientists transplanted BEM-based iN cells into an ischemic stroke-induced adult mouse model of hypoxic brain damage. In order to observe their therapeutic potential. In addition to neurogenesis, the paracrine effects of iN cell transplantation restored the motor coordination and mobility skills of these mice, demonstrating their therapeutic effects.
Interestingly, cells cultured on 3D BEM hydrogels showed higher levels of neuronal marker expression compared to 2D BEM, suggesting that brain-like bionic microenvironments provide optimal biochemical and biophysical signals. To guide cell reprogramming. The researchers then cleverly combined the three-dimensional BEM hydrogel with a microfluidic system (3-D μBEM) to expand the autocrine and paracrine signals of iN cells in a restricted microenvironment, thereby promoting neuronal transduction differentiation.
When iN cells generated by reprogramming in a brain-like 3-DμBEM environment were collected and transplanted into a mouse model of ischemic stroke, the researchers observed functional recovery and therapeutic efficacy. To clarify the molecular mechanisms involved, scientists have suggested that biophysical cues in a three-dimensional brain-like microenvironment may specifically induce the localization of YAP into the cytoplasm, thereby promoting neuronal transdifferentiation. In contrast, as observed in this study, a hard two-dimensional environment induces the localization of YAP into the nucleus, thereby inhibiting gene expression associated with neurogenesis.
This study reveals the molecular mechanisms of direct neurogenesis, as well as the design and development of optimized biomaterials to improve neural reprogramming strategies to reproduce this neuronal reprogramming in the laboratory. The brain-like three-dimensional biomimetic microenvironment provides biochemical, biophysical, mechanical, and epigenetic stimulation to effectively reprogram neurons.
The main findings of this research can be transformed in the future to design synthetic brain-like matrices that produce safe iN cells for regeneration and medical applications in neuroengineering.